Our team has aimed to create a transportation method capable of a 5-kilometre-long round trip on Ganymede, one of Jupiter’s moons. This vehicle must handle extreme conditions including temperatures as low as -180C, low gravity(a tenth of Earth’s), rocky terrain, and interference from the planet’s magnetic field affecting electronics.
In this project, I oversaw the chassis’s “design.” We stripped down an existing RC car, removing unnecessary parts and adding new ones.
Method/Procedure:
- Brainstormed and created a definition statement
- Brainstormed different designs and features for the vehicle including:
- 4 Axle Design
- 6 Wheel Design
- Hybrid Powertrain
- Full Electric Powertrain
- Full Gasoline Powertrain
- Thrusters on the top to compensate for low gravity
- Proposed Design for Vehicle
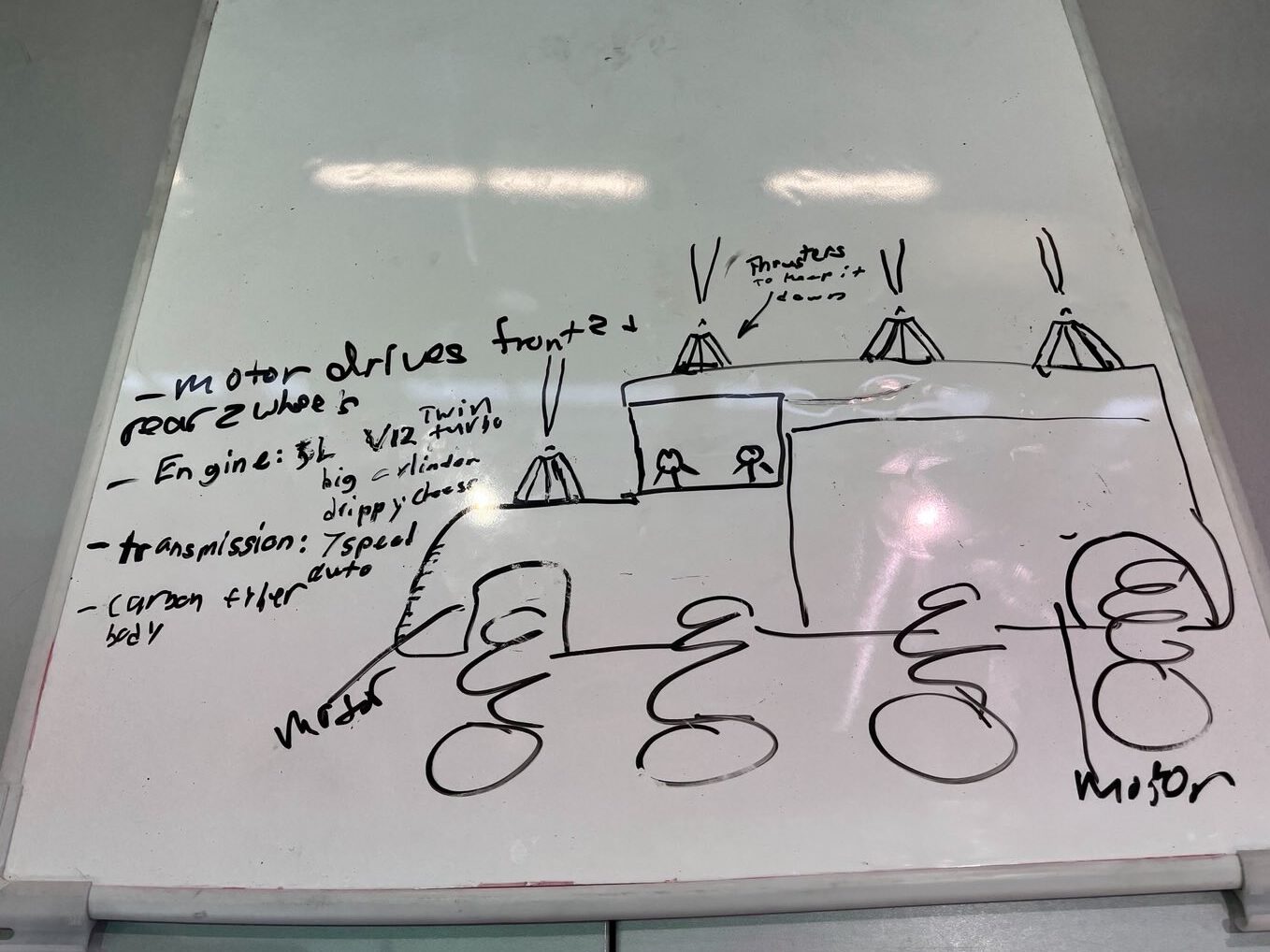
In the end after our brainstorming, we found that it would be difficult to implement so many features in such a short timeframe as well as in such a small example. We decided to go with a Hybrid powertrain driving 6 wheels, using a small supercharged 3l Inline 6 powering the rear 4 wheels, and 2 electric motors to drive the front 2 wheels individually allowing for torque vectoring on unknown terrain. The decision to go for a supercharger was so that even in low oxygen atmospheric conditions, it forces more air into the combustion chambers, maintaining near-earth power output as well as fuel efficiency purposes, because more oxygen in the intake charge allows for more complete fuel combustion, reducing issues like incomplete combustion, rough idling, and excessive fuel consumption.
The decision to use 2 electric motors at the front was for backup purposes, as well as fuel efficiency purposes, allowing for the engine to charge the batteries for the motors in addition to limited solar power.
We also opted for air suspension for added comfort and stability on this terrain. Tires would be a soft compound for the ability to traverse over rocky and icy terrain with spikes.
Prototype Model/Proposed Design
Final CAD Model

Testing
For testing, we were unable to get access to any type of engine that would fit in our vehicle let alone a supercharger that would fit, so we kind of “ignored” that part of the vehicle although it would be very important to test that if we had the access to an engine or a larger model as well as a testing environment to reduce atmospheric pressure and oxygen. We also would have added our thruster idea for gravity stability in low-gravity environments.We ultimately decided to use rocks and gravel, which we scattered on a tarp. Since it was also cold that day, the sand and gravel were frozen, mimicking the surface of Ganymede—rocky ice. We formed different-sized hills and split them into three categories: small, medium and large hills as well as obstacles for the vehicle to traverse. If given access to more equipment and resources, testing would include extremely negative temperatures and radiation testing. We tested:
- Time
- Speed
- Ability to traverse the terrain
- Efficiency

We calculated:
v = velocity 0.835m/s (3km/h)
KE≈0.3486J
Data Examples
(If graphs are too small to read, please right-click to open image in a new tab)
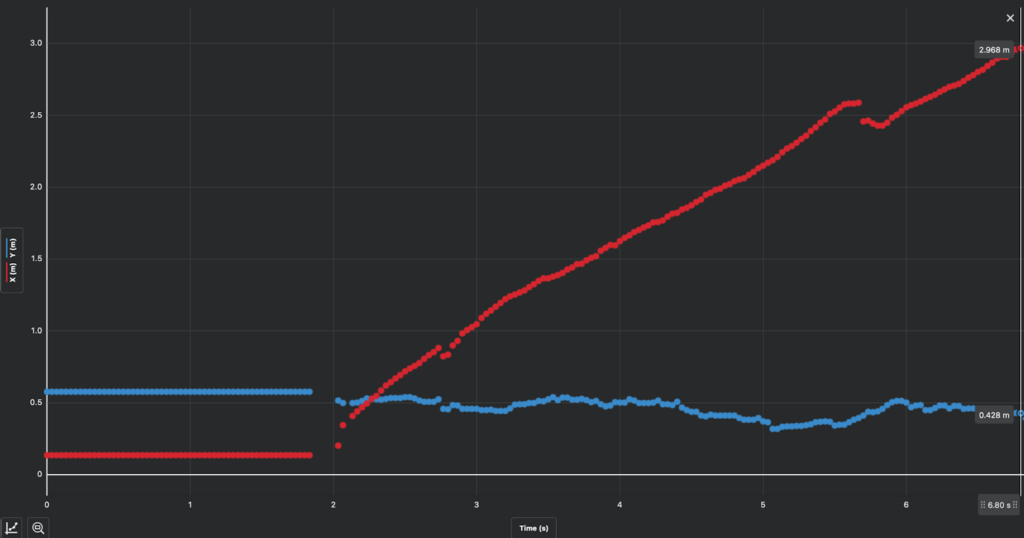
Test 6 (Medium)
6.83 Seconds
Velocity: 0.4002200943 m/s
Total distance: 2.968 meters
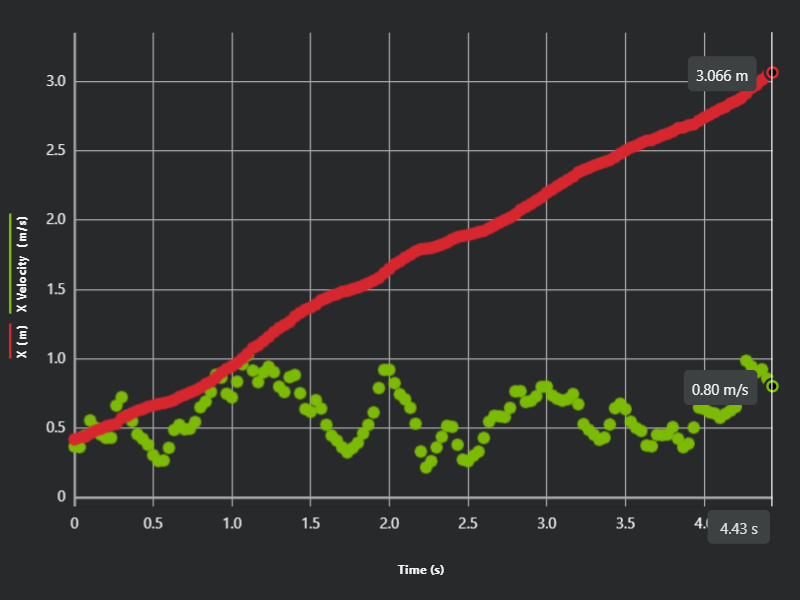
Test 5 (Medium)
4.43 Seconds
3.066 Meters
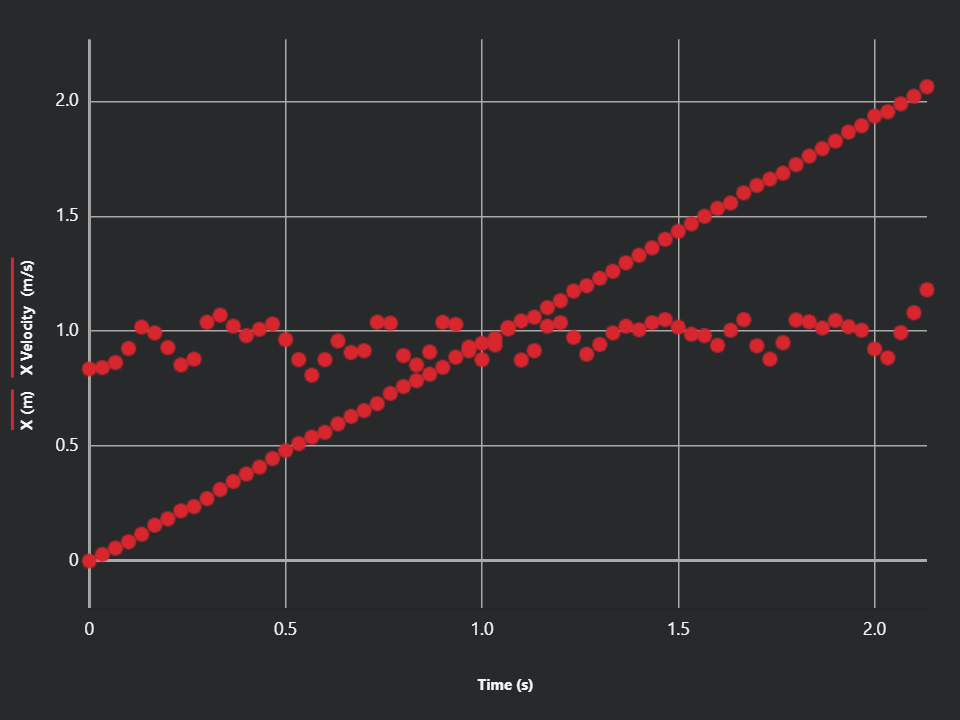
Video 2 (Control)
2.13 Seconds
Velocity: ~0.9m/s
Total distance: 2.064 meters
Video 8 (Hard)
3.9 Seconds
0.557m/s
Total Distance: 2.172m
Analysis
Firstly, we need to calculate the control tests’ average time.
The equation for the control tests are:

As speed is the distance over time, the average speed is the average distance over average time. Let’s calculate the control tests’ average speed.
Since speed is distance over time, the average speed is avg distance/avg time.

To calculate the average velocity for all three “easy, medium and hard” tests we did we need to use the same equation.
Easy:

Medium:

Hard:

Control Tests (Videos 1 and 2):
The control tests are pretty consistent and showcase the vehicle’s ability to drive over the “ideal” terrain. The average speed serves as a benchmark for comparing the other tests, representing the “maximum”/”ideal” speed achievable.
Easy Tests (Videos 4, 5):
A noticeable reduction in speed occurs since the vehicle is required to cover a longer distance, rather than the terrain directly restricting its speed. Terrain A offers moderate difficulty, primarily emphasizing maneuvering around craters. While these craters are not scaled to size, they serve as obstacles symbolizing larger features like mountains, boulders, and or other challenges. The vehicle was mainly driven around the obstacles in order to achieve the most efficient route possible.
Medium Tests (Videos 7, 8):
The tests on Terrain B demonstrate a greater level of difficulty, as indicated by the variation in time and distance. This terrain features additional complex elements, such as larger obstacles and steeper inclines. Unlike previous tests, we drove the vehicle directly over these challenges rather than around them to test real maneuverability compared to the most efficient route. The vehicle performed quite well in these conditions, even surpassing the average speed achieved in the easier maneuverability tests. This success is likely due to the synergy of the vehicle’s suspension, traction, and maneuverability working together effectively.
Hard Tests (Videos 6, 9, 10):
The hard terrain by far the most complex to traverse, primarily stressing and putting emphasis the vehicle’s traction and suspension. The vehicle did struggle to maintain speed and traction a bit, as evidenced by a significant drop in average velocity compared to earlier tests. Steep inclines and uneven surfaces present considerable challenges, causing the tires to slip at several points throughout the test. To improve performance in such demanding conditions, enhancements in suspension and stability features, such as a roll cage and electronic traction control will probably benefit the vehicle if this is brought to Ganymede.
Hard Test (Big Hill) (Video 10):
The Big Hill test took the longest, which we anticipated due to the steep incline that caused the vehicle to tip over and lose stability. This performance underscores the need for specific improvements to better handle steep inclines, ensuring the vehicle can navigate such terrains safely and efficiently.
The equation for efficiency:

Efficiency for all tests:

Medium:

Hard:

Conclusion
In conclusion, the vehicle we tested proved capable of traversing various terrain to a certain extent. It was extremely competent in traversing rocky, sandy gravel terrain and capable of traversing over small + medium hills. A challenge that occurred, however, was traversing over sandy and rocky large hills. The tyres would often slip and lose traction on the hill, compromising stability and agility. If there were to be a future redesign with more resources and more time, it would be helpful to add systems like ESC (Electronic Stability Control), Traction control, and a locking differential on both axles. Adding these issues would address the issues presented.
AI Disclosure
AI was used for efficiency equations here
Leave a Reply